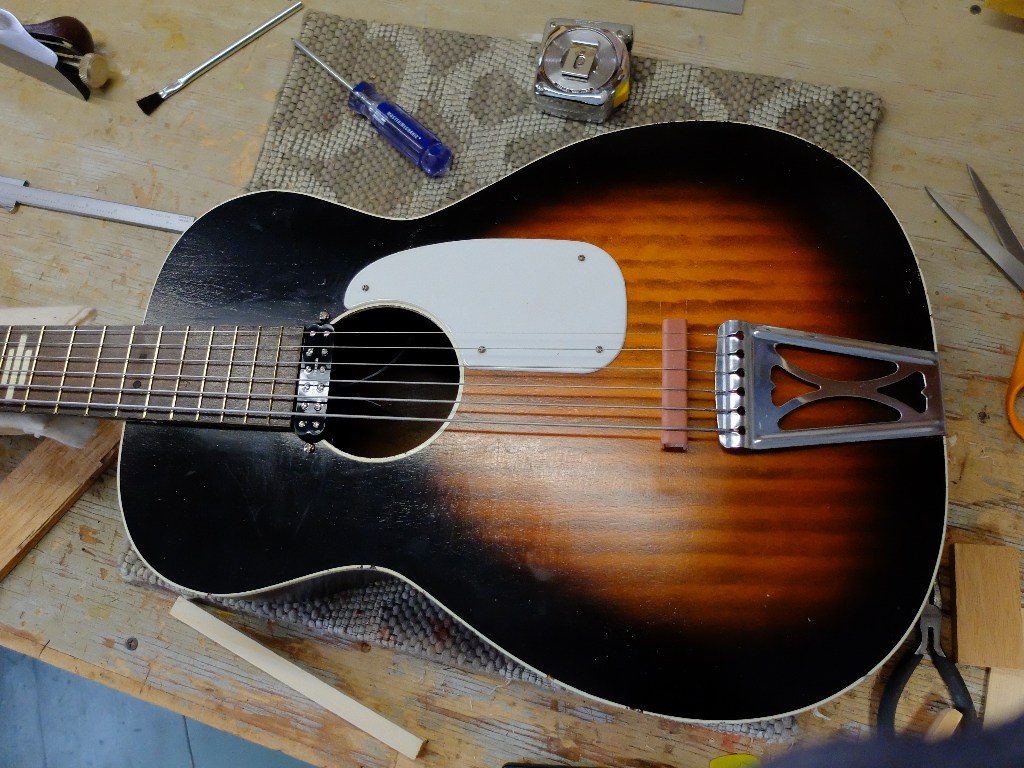
This blog is a continuation of two previous blogs starting with the Stella rubber bridge conversion pictured above. My impression of the Stella/Harmony guitar had a serious impact on me: it went pretty easily, and I therefore expected easy success in replicating this guitar, and the quality of the Stella built encouraged cavalier handling of lutherie ethics which were up to this point sacred ground for practicing builders. I set out immediately to build one from scratch, ignoring all my accumulated knowledge about using only quarter-sawn lumber, light weight top material – spruce of red cedar – for tops, tap-tuning, etc. This one is made from flat-sawn cherry, complete with bark inclusions, and thicknessed to about 0.150″. Inside of top: Heavy braces with layout and dimensions copied from Stella.
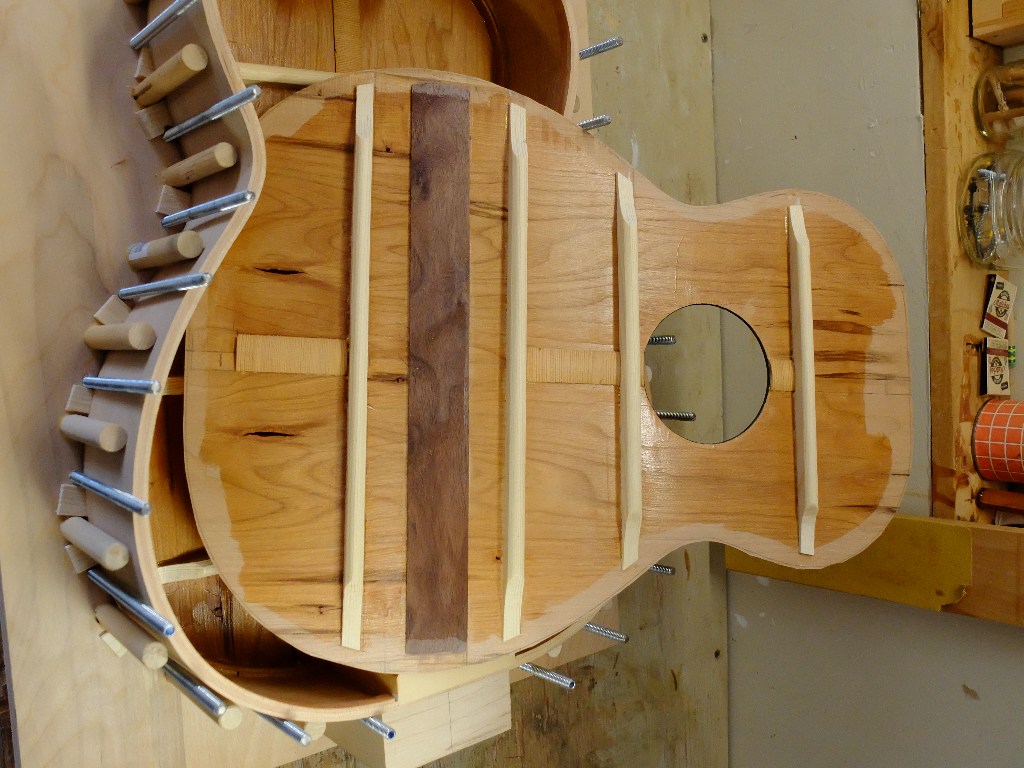
I did take the precaution of giving the insides a seal-coat of shellac in an anticipation of controlling dimensional change with humidity which the flat-sawn lumber should be more reactive to than quarter-sawn.
I could not find other documentation of Stella bracing, except this – which reinforced my impression that resonance was not a feature necessary for this instrument – 3 top braces at 1/2 x 3/4″ with a couple massive flat braces
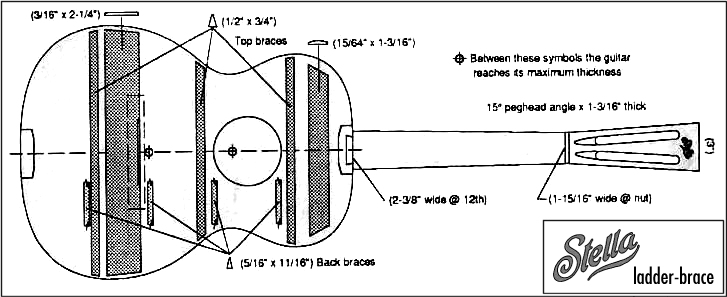
The back and sides of this new instrument are also cherry, quarter-sawn for the sides, but flat-sawn back. Here is the back ready to be glued on, with the access hole already cut. The top is on the left. I do use bent solid wood lining which takes a little more effort than the store-bought kerfed stuff – I think the end result is superior, and I can’t stand to pay $24 or something for the kerfing.
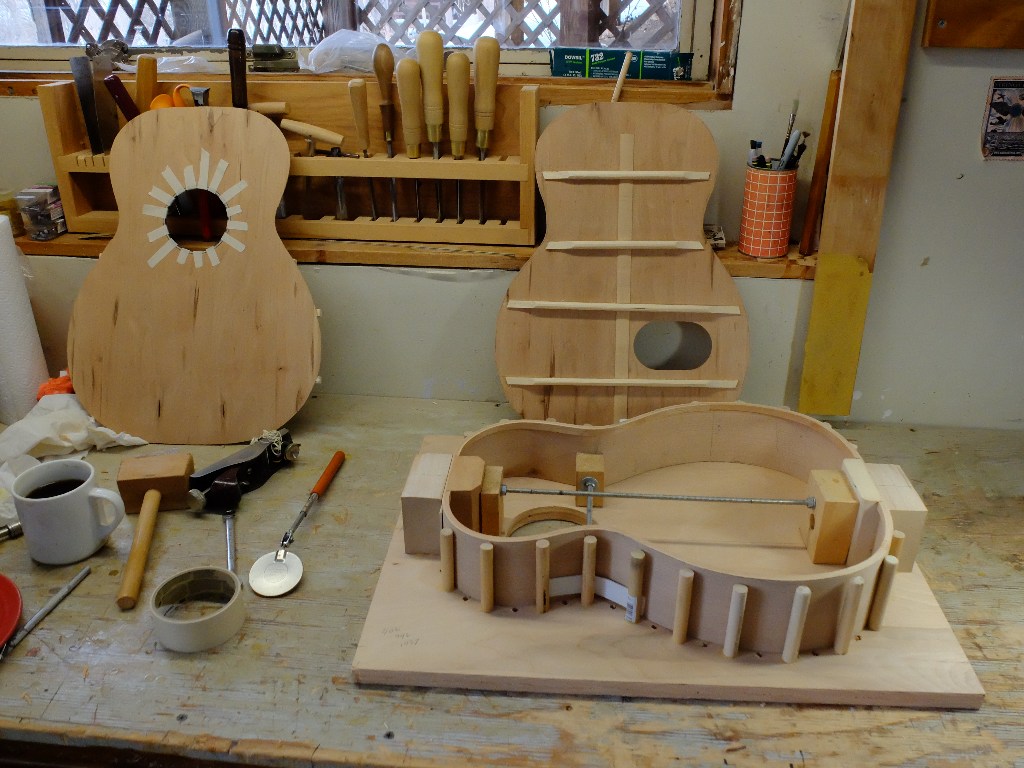
This instrument turned out great too, like the Stella conversion. I used the same pickups – Seymour Duncan Little 59 Strat mini-humbucker at the neck and the K&K Big Shot piezo under the bridge. Like the Stella, it took several trial placements before finding the best spot for the piezo, and I had to use the very thin double-sided tape that comes with the K&K for the attachment instead of the 3M foam used on the Stella, but it worked.
Success #2. Look for this one on stage if you are at a Guster concert. I thought I had it down. I accepted an order…
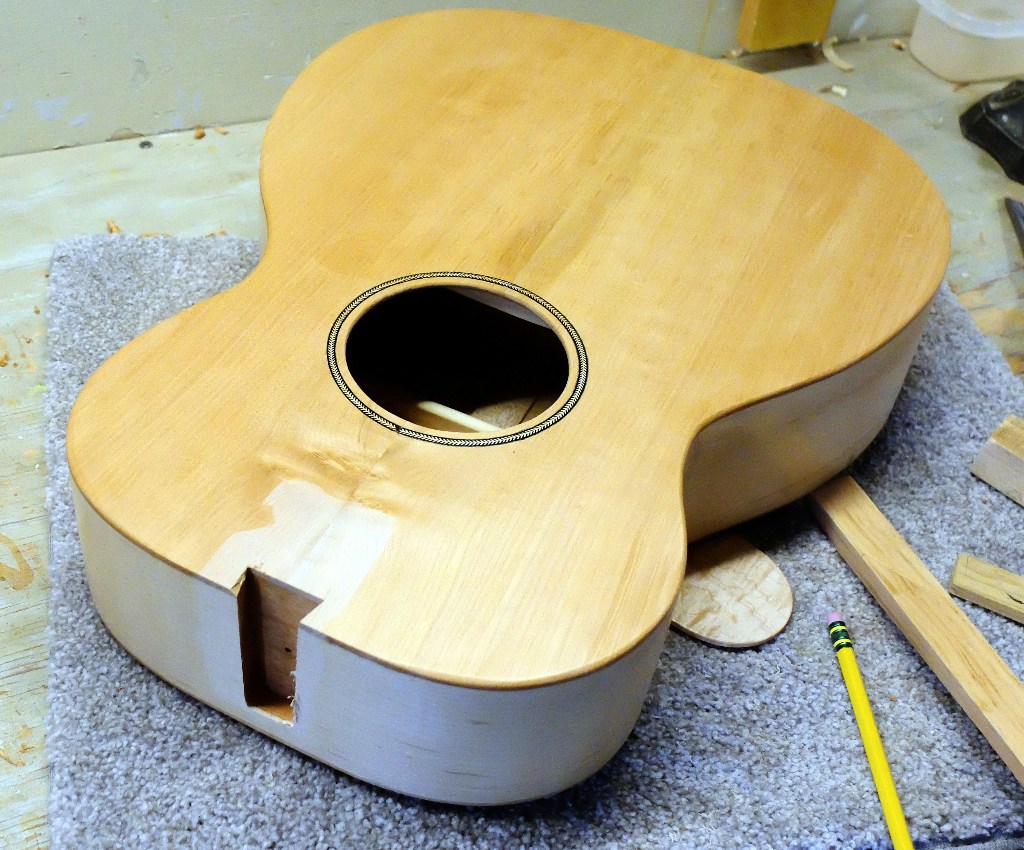
Building on the (now-mistaken) idea that resonance didn’t matter, I made the next one out of maple. Nice quarter-sawn back and sides, and flat-sawn big-leaf maple top. I used the Stella bracing dimensions.
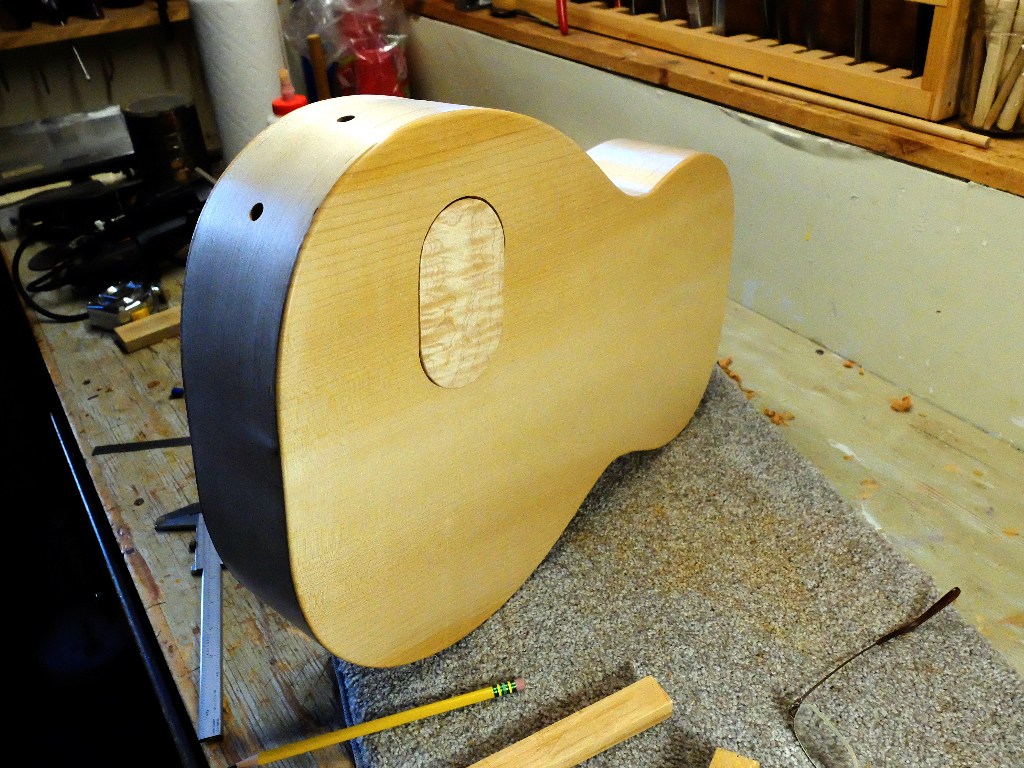
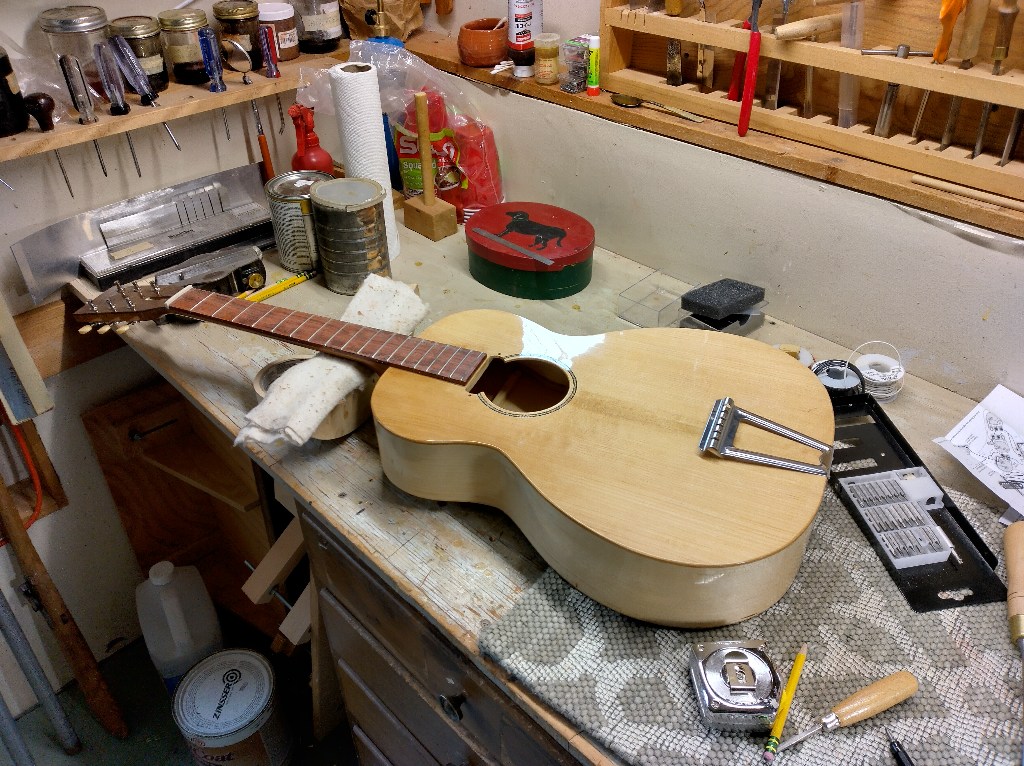
And it didn’t come out – that is, I couldn’t get the piezo to produce a signal that even came close to matching the humbucker. It was too dead. I switched to a dual-humbucker configuration wired like a Telecaster, but with a toggle that can send the bridge pickup out its own jack (I had to use the second output jack for something). Success, but not what the customer ordered. I refunded the deposit check, thought I had learned what it takes, and built another.
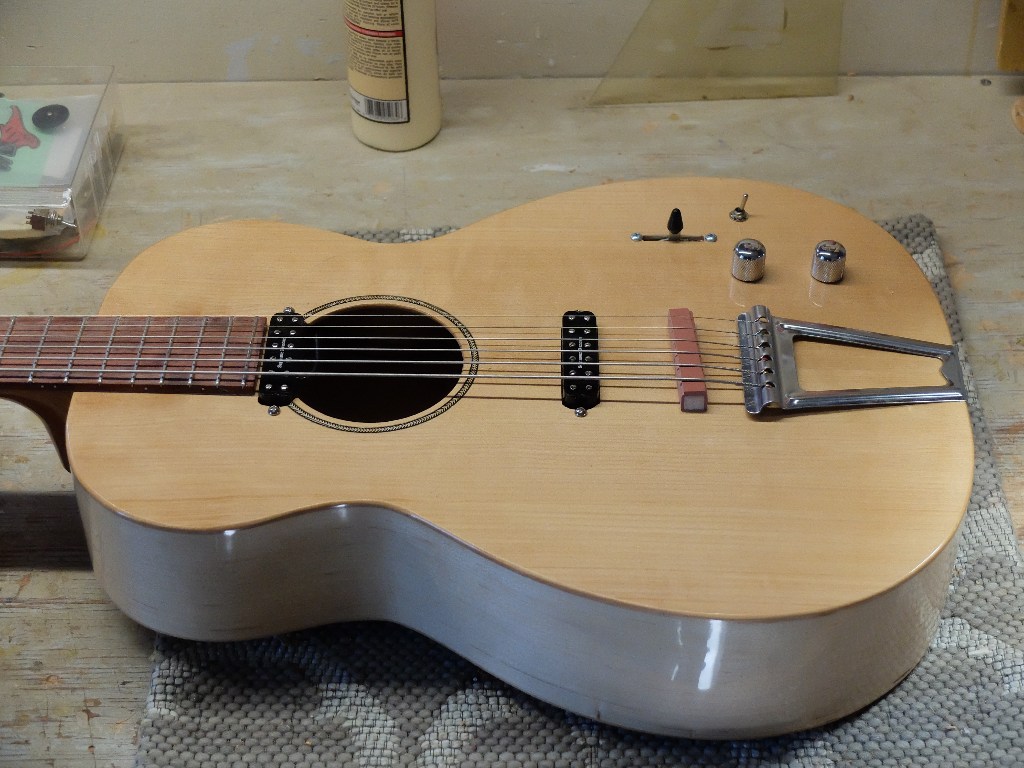
This next one has a spruce top, although a rather funky specimen from a tree that came out of somebody’s yard in Shelburne Vt about 15 years ago that I got from Roger Borys. I thinned the top braces and used spruce for the under-bridge reinforcement.
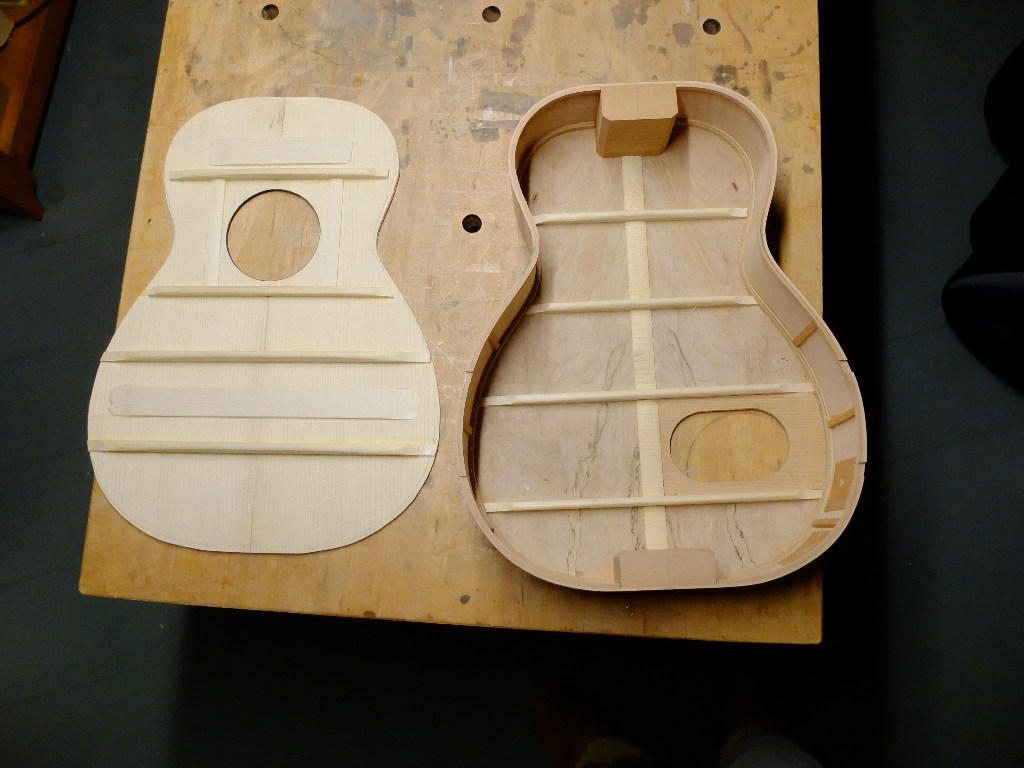
The back is a nice piece of spalted/quilted/curly big-leaf maple from Oregon
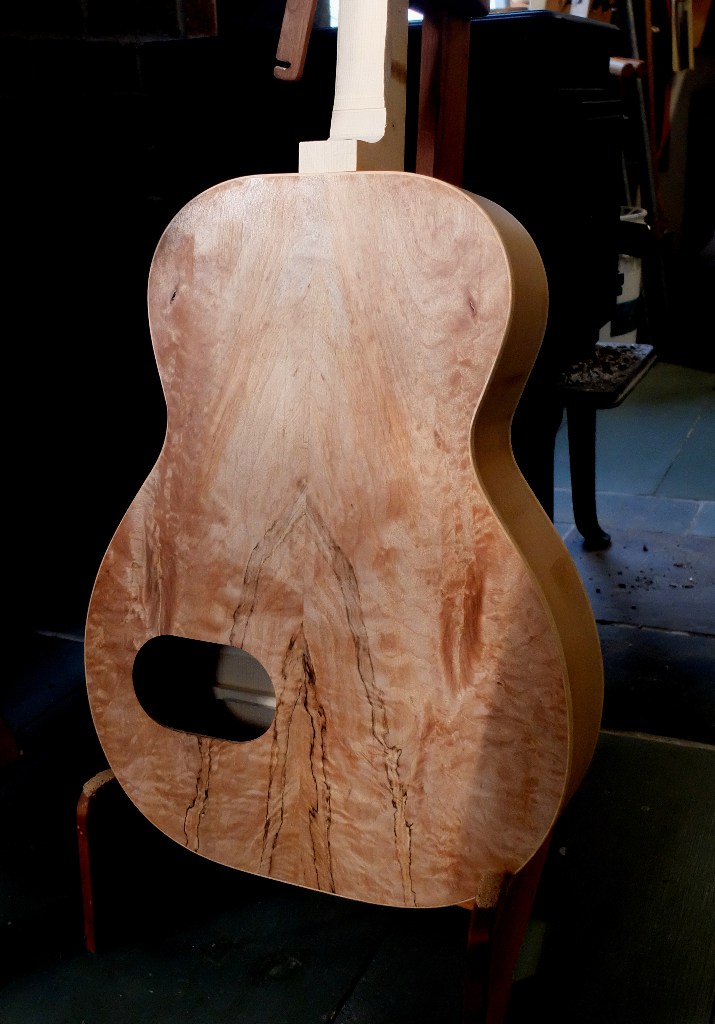
Cut to the chase – this didn’t turn out either. Piezo a no-go. I did the same dual-humbucker setup as the first ‘failure’, but this time moved the bridge pickup closer to the bridge for more bite, which necessitated raising the pickup in a nice little ebony mounting ring to get even balance between the two humbuckers. I now have in my inventory two interesting examples of rubber bridge guitars in a 3/4 size that have a lot of personality and attitude. Not a bad problem to have. I am trying again.
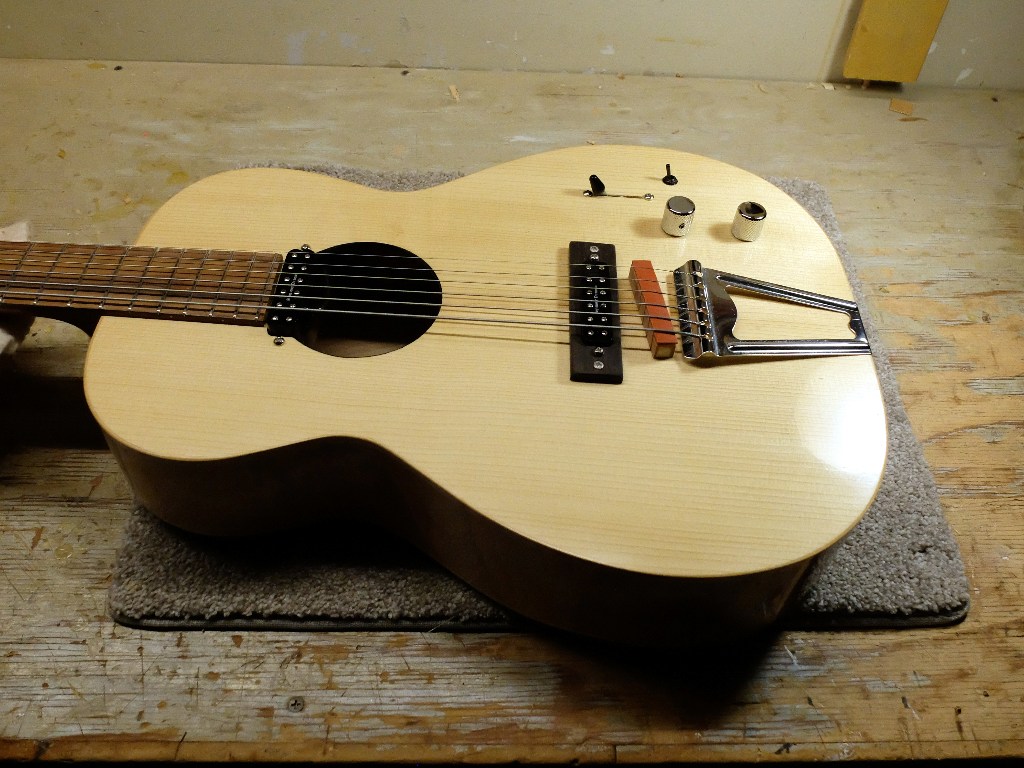
This one is a no-nonsense acoustic instrument called ‘The Hope’. I used real European spruce for the top (from Metropolitan Music in Stowe) and an ‘X’ brace pattern. The back and sides are cherry, quarter-sawn. The top has ring to it. It is in the final finishing stages and I will be able to string it up in about 2 weeks. I will post the results.
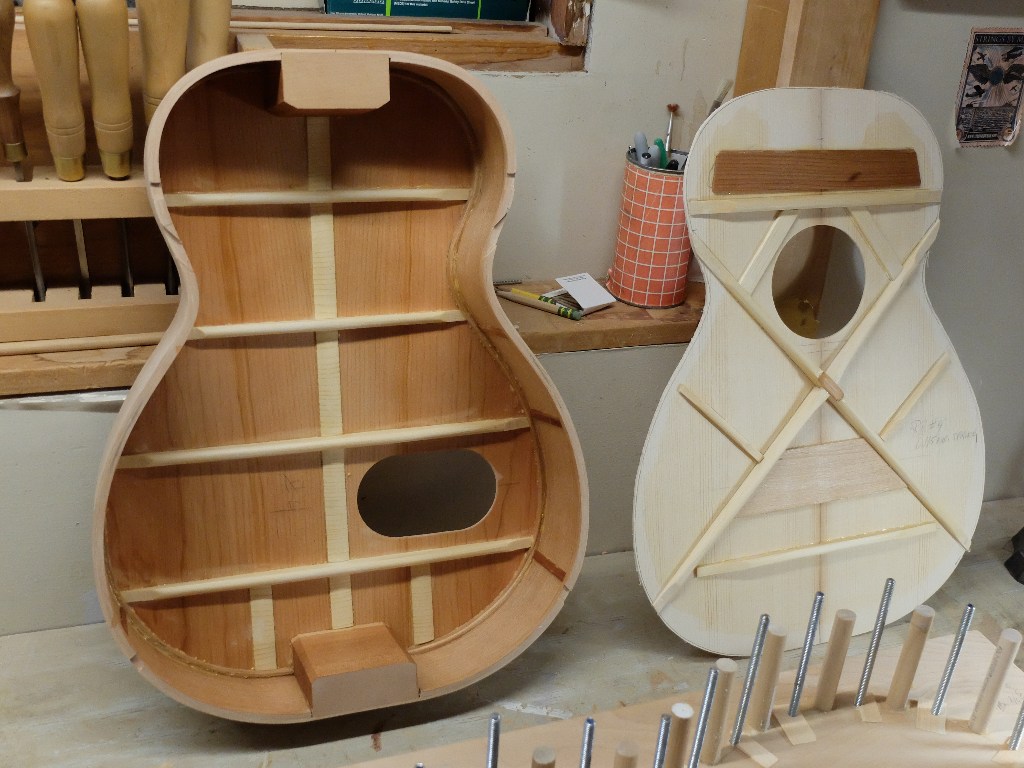
Results – I have to accept the fact that a piezo pickup cannot match the output of a magnetic pickup without a preamp. Almost all references to piezo’s recommend a preamp. This guitar, ‘The Hope’, worked with the piezo, but the signal was nowhere near balanced with the output of the Seymour Duncan magnetic. So – I abandoned the piezo, not being interested in a lot of tweaking to come up with a janky piezo sound, and just went with the single magnetic pickup with volume/tone controls. The result is an elegantly clean-looking instrument with lots of tonal capability and ready playability without a lot of gear messing around.
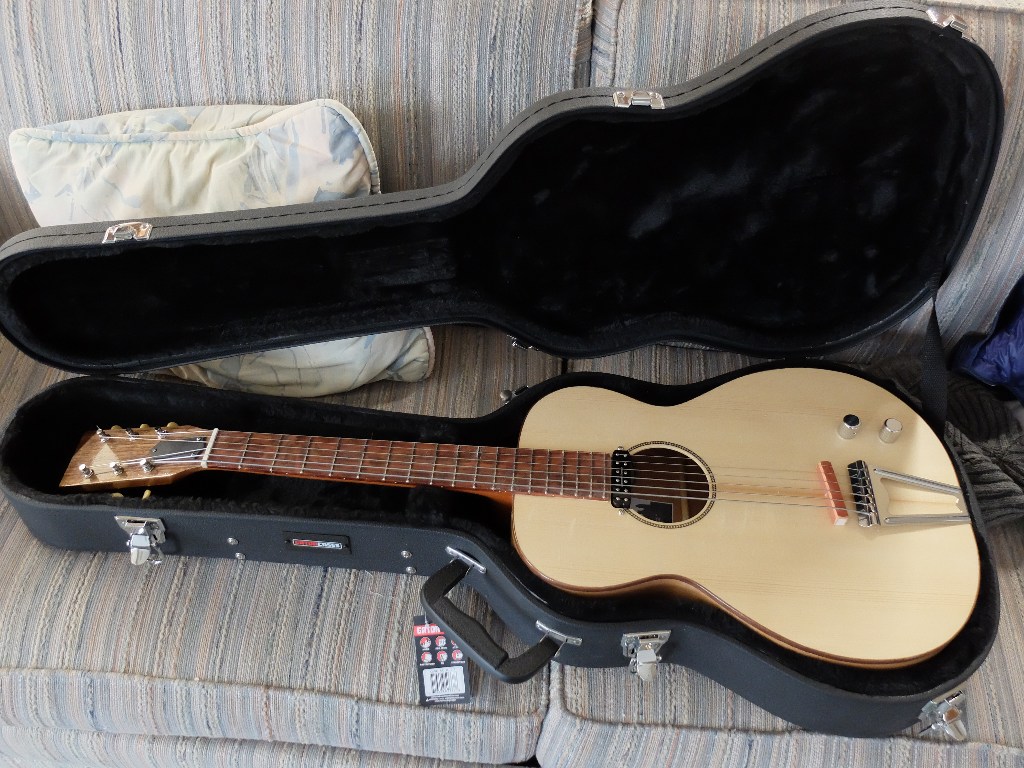